Principles of Acoustic Design for Room Acoustics
- James
- Mar 4
- 7 min read

When designing acoustics for a room, the objective is to optimise the behaviour of sound waves, ensuring clarity or privacy, comfort, and performance. Achieving this requires more than just intuition; it involves an understanding of acoustical principles and the application of various models and calculation methods. In this article, we’ll explore advanced principles of acoustic design and introduce methods like the Sabine and Eyring equations, which are central to optimising room acoustics.
The first and most crucial step in any acoustic design process is understanding the specific requirements of the space in question. Acoustic needs can vary dramatically based on the intended function of the room, and identifying these needs early on can ensure that the appropriate treatments and design strategies are implemented. Spaces are often incorrectly thought of as having ‘good’ or ‘bad’ acoustics. One fundamental question to ask when assessing a space is whether privacy or clarity is the primary acoustic requirement.
Privacy vs. Clarity: Defining the Acoustic Requirements
Privacy
Privacy is often a key concern in spaces where confidential or personal conversations take place. For example, in a restaurant, patrons may want to have a quiet meal without the distraction of overhearing neighbouring tables' conversations. Similarly, offices, medical clinics, and meeting rooms also need sound isolation to prevent sensitive discussions from being unintentionally overheard. In these cases, achieving isolation becomes paramount. The goal is to block external sound from entering the room while ensuring that the sound produced within the space doesn't escape and disrupt surrounding areas.
Effective acoustic privacy can be achieved through the use of sound-absorbing materials, such as acoustic panels, carpet, and fabric wall coverings, to minimise sound reflections or reverberation. Furthermore, soundproofing techniques such as decoupling walls, using resilient channels, or installing double-glazed windows help to reduce sound breaking in or out of a room. Ensuring that these treatments are designed and placed correctly is essential to maintaining a balance between usability and discretion in spaces where privacy is critical.
Clarity
On the other hand, clarity is the primary concern for spaces where clear, intelligible speech or sound reproduction is essential. This includes environments such as auditoriums, cinemas, lecture halls, conference rooms, and even open-plan offices. In these spaces, ensuring that the speech is easily understood or that sound is accurately conveyed is of the utmost importance. For example, in an auditorium or cinema, the clarity of speech or dialogue is vital to the audience’s experience, and poor acoustics can lead to frustration and misunderstandings.
Clarity requires a well-thought-out combination of acoustic treatments that optimise sound reflection and absorption. The goal is to manage reverberation time (the time it takes for sound to decay after it has been produced) so that the sound remains clear without being muddled by excessive reflections. This is typically achieved through the careful placement of absorptive materials like acoustic panels and materials that reduce reverberation. Moreover, room dimensions and shape must be considered to prevent issues such as focusing or flutter echoes, where certain frequencies are amplified due to the room's geometry.
In spaces where speech is critical, such as classrooms or meeting rooms, clarity can be further enhanced by incorporating directivity control, such as using directional speakers or strategically placing microphones to ensure that the sound is focused where it’s needed most.
Balancing Privacy and Clarity
Interestingly, some spaces require a balance of both privacy and clarity. For instance, an open-plan office might need to ensure speech intelligibility in collaboration areas while also maintaining sufficient privacy to avoid distractions and confidential conversations from being overheard. In such cases, a combination of both sound absorption (to reduce reverberation and improve clarity) and sound isolation (to block unwanted noise from entering or leaving the space) will be required. In some of these office layouts, acoustic booths or pods have become popular.
Understanding whether privacy or clarity is more critical, or whether a balance of the two is needed, serves as the foundation for all further acoustic design decisions. Once these priorities are established, the design process can then proceed with the selection of appropriate materials, technologies, and layout configurations to meet the specific acoustic needs of the space.
1. Room Size and Shape: Influence on Acoustic Behaviour
The size and shape of a room have a profound effect on how sound propagates, and this can be quantified using models like the Sabine and Eyring equations. The dimensions of the room contribute to the formation of standing waves and resonance, which need to be controlled for accurate sound reproduction.
Room Modes and Resonance
Room modes are the frequencies at which standing waves occur due to the room’s dimensions. For instance, the length of a room can support a certain number of low-frequency standing waves. These resonances create uneven frequency responses, and careful planning can minimise their effects. For instance, if you were designing a cinema or listening room, it would be desirable to position seats away from significant peaks or troughs in frequency response.
2. Reverberation Time: Sabine and Eyring Equations
One of the central aspects of room acoustics is controlling reverberation time, which is the time it takes for sound to decay in a room after the sound source stops. Too much reverberation can lead to unclear speech or muddled music, while too little reverberation can make the room sound "dead" or overly dry.
Sabine Equation
The Sabine equation is the most commonly used formula for estimating the reverberation time (RT60) of a room. It is based on the assumption of a uniformly distributed sound field and a well-mixed room.

Where:
RT60 is the reverberation time in seconds,
V is the volume of the room in cubic meters,
A is the total absorption in the room, measured in sabins (the product of the absorption coefficient and the surface area).
The Sabine equation assumes that sound is equally absorbed across all surfaces and that the absorption properties are constant. It’s useful for estimating reverberation in spaces like concert halls or auditoriums, where the sound field is generally diffuse.
Eyring Equation
While the Sabine equation is widely used, it becomes less accurate in rooms with high absorption, especially when the room has a large surface area with highly absorptive materials. The Eyring equation is more accurate in such scenarios and is often applied when absorption is high (e.g., rooms with a lot of carpeting, acoustic panels, or thick curtains).
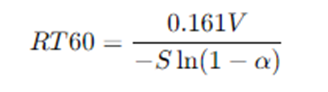
Where:
α the average absorption coefficient of the surfaces,
S is the surface area of the room.
The Eyring equation accounts for the fact that as absorption increases, the sound field becomes less diffuse, and the reverberation time decreases more quickly than predicted by Sabine’s model. This equation is particularly useful in spaces that have extensive sound-absorbing materials like recording studios or lecture halls.
3. Absorption and Reflection Control: Quantifying Treatment Effects
The key to creating a balanced acoustic environment is achieving the right mix of sound absorption and reflection. Absorptive materials reduce reverberation time and prevent excessive echoes, while reflective surfaces can enhance certain frequencies. However, precise control of these factors is essential.
Absorptive Materials
Absorption coefficients (α) quantify how much sound a material absorbs at different frequencies. Materials such as acoustic panels, carpets, and curtains generally have good absorption coefficients, meaning they absorb a significant proportion of sound energy. However, the absorption rate can vary depending on the frequency of the sound.
For example, low-frequency sound waves tend to be more difficult to absorb, requiring specialized treatments like bass traps or thicker materials with greater depth. To predict how these materials will affect the acoustics of a room, one must consider both the absorption coefficient and the total surface area of the material.
Surface Area and Distribution
The total absorption in a room can be calculated by summing the absorption coefficients of various materials multiplied by their surface areas. This allows for accurate predictions of how much sound energy will be absorbed and how this affects the overall reverberation time. Proper placement of absorptive materials is also crucial—strategically placing them at reflection points (such as first reflection points on walls) can help reduce sound distortion and improve clarity.
4. Bass Management: Low-Frequency Control
Bass frequencies (below 100 Hz) are particularly challenging to manage due to their long wavelengths, which cause them to accumulate in corners and other areas of the room. Effective bass management involves understanding the behaviour of these low frequencies and applying targeted treatment.
Bass traps are typically placed in corners, where low-frequency sound waves tend to accumulate. These traps work by using materials that absorb sound at lower frequencies, thereby reducing the peak responses that can cause boominess or muddiness in the room. The effectiveness of bass traps is typically measured by the frequency range they absorb and their placement within the room.
5. Sound Isolation and Noise Control
Sound isolation plays an integral role in preventing external noise from infiltrating a room and ensuring sound doesn’t escape to other areas. This is particularly important in recording studios, home theatres, or any environment where sound privacy is crucial.
6. Diffusion: Ensuring Even Sound Distribution
Diffusion is the process of scattering sound waves in various directions, preventing excessive direct reflections from creating acoustic hot spots. Diffusers work by breaking up the sound wave’s energy, redistributing it evenly throughout the space.
Diffuser Design
Diffuser design can be quantified through the Schroeder diffusion coefficient, which measures the uniformity of sound distribution. Good diffusers should scatter sound evenly across frequencies, especially in rooms intended for music or performance. The placement of diffusers is calculated based on the size of the room and the listening area, with specific attention paid to critical reflection points.
In conclusion, achieving the right acoustic environment depends on understanding the primary needs for your space. By carefully managing reverberation, and sound insulation optimal acoustic performance can be achieved. At JG Acoustics Ltd, we offer expert guidance and design advice to help meet your unique acoustic requirements. Contact us today to discuss your project, and let us help you create the ideal acoustic setting for your space.
Material | Absorption Coefficient (0.125 kHz) | Absorption Coefficient (0.250 kHz) | Absorption Coefficient (0.500 kHz) | Absorption Coefficient (1.000 kHz) | Absorption Coefficient (2.000 kHz) |
Concrete (smooth) | 0.03 | 0.04 | 0.05 | 0.08 | 0.12 |
Concrete (rough) | 0.07 | 0.08 | 0.09 | 0.11 | 0.14 |
Brick | 0.07 | 0.08 | 0.1 | 0.12 | 0.16 |
Wood panel | 0.15 | 0.2 | 0.25 | 0.35 | 0.45 |
Glass (single) | 0.05 | 0.1 | 0.15 | 0.2 | 0.3 |
Glass wool | 0.9 | 0.95 | 0.98 | 0.99 | 1 |
Fiberglass insulation | 0.85 | 0.9 | 0.95 | 0.97 | 0.99 |
Carpet (short pile) | 0.2 | 0.3 | 0.4 | 0.5 | 0.6 |
Carpet (long pile) | 0.5 | 0.6 | 0.7 | 0.8 | 0.9 |
Cork | 0.25 | 0.4 | 0.5 | 0.6 | 0.7 |
Curtain fabric | 0.5 | 0.6 | 0.7 | 0.8 | 0.9 |
Plasterboard | 0.05 | 0.1 | 0.15 | 0.2 | 0.25 |
Acoustic foam (high density) | 0.9 | 0.95 | 0.98 | 0.99 | 1 |
#AcousticDesign #RoomAcoustics #SoundClarity #AcousticSolutions #PrivacyInDesign #AcousticConsulting #NoiseControl #Reverberation #SoundAbsorption #EyringEquation #SabineEquation #AcousticTreatments #SpaceDesign #JGAcoustics #AcousticExpert #AcousticOptimisation #RoomSoundDesign #BuildingAcoustics #SoundManagement #NoiseReduction #SoundInsulationTesting #NoiseImpactAssessments #BS4142 #NoiseAssessment #BuildingSoundTesting
Σχόλια